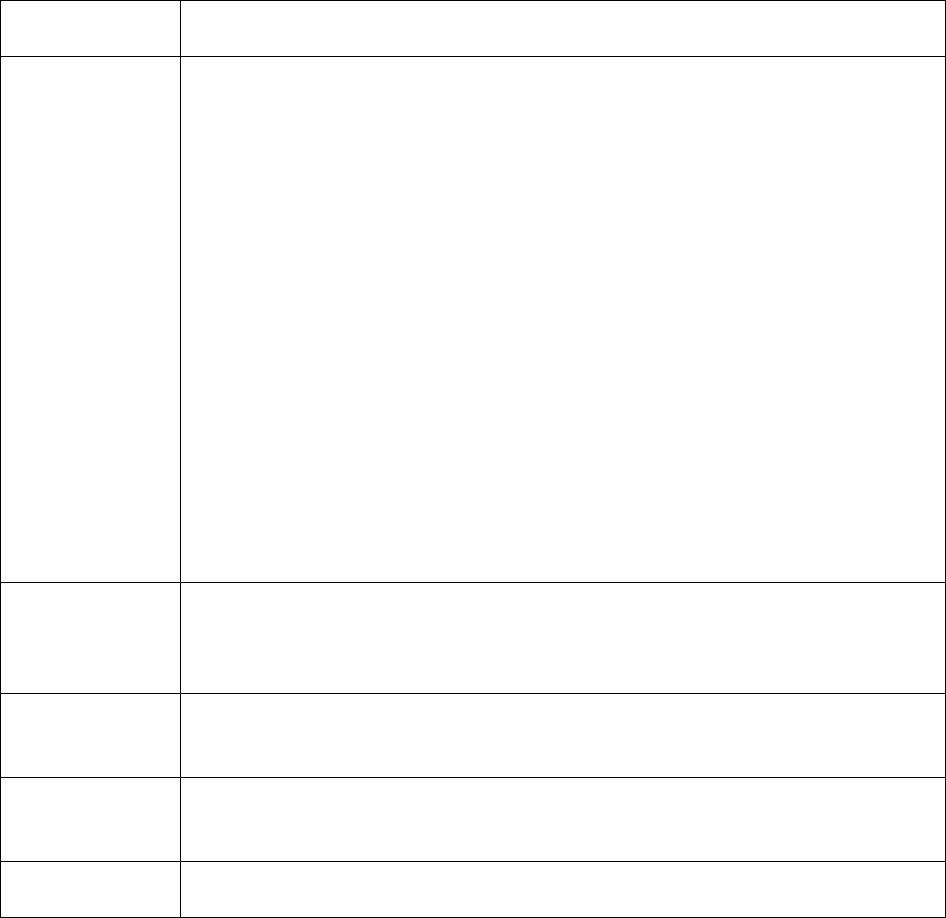
53
Table A2. Zucker-Darby S&E Field Categorization and ISI Category Descriptions
Zucker-Darby
Categorization
ISI Journal Category Description
Biology/
Medicine/
Chemistry
Agriculture/Agronomy, Anesthesia & Intensive Care, Animal & Plant
Sciences, Animal Sciences, Neurosciences & Behavior, Biochemistry &
Biophysics, Biology, Biotechnol & Appl Microbiol, Cardiovasc & Respirat
Syst, Cell & Developmental Biol, Oncogenesis & Cancer Res, Agricultural
Chemistry, Chemical Engineering, Chemistry & Analysis, Chemistry,
Cardiovasc & Hematology Res, Dentistry/Oral Surgery & Med,
Dermatology, Medical Res, Diag & Treatmt, Endocrinol, Nutrit & Metab,
Entomology/Pest Control, Environment/Ecology, Experimental Biology,
Food Science/Nutrition, Gastroenterol and Hepatology, General & Internal
Medicine, Hematology, Immunology, Inorganic & Nucl Chemistry, Clin
Immunol & Infect Dis, Molecular Biology & Genetics, Microbiology,
Resrch/Lab Med & Med Techn, Medical Res, General Topics, Neurology,
Endocrinol, Metab & Nutrit, Medical Res, Organs & Syst, Oncology,
Ophthalmology, Organic Chem/Polymer Sci, Orthopedics & Sports Med,
Otolaryngology, Pediatrics, Physical Chem/Chemical Phys, Pharmacology &
Toxicology, Plant Sciences, Pharmacology/Toxicology, Psychiatry,
Physiology, Clin Psychology & Psychiatry, Radiol, Nucl Med & Imaging,
Reproductive Medicine, Rheumatology, Environmt Med & Public Hlth,
Surgery, Urology, and Veterinary Med/Animal Health
Computer/
Information
Processing/
Multimedia
AI, Robotics & Auto Control, Computer Sci & Engineering, Engineering
Mathematics, Info Technol & Commun Syst, and Mathematics
Integrated
Circuit/Semi- &
Super-conductor
Appl Phys/Cond Matt/Mat Sci, Elect & Electronic Engn, Mechanical
Engineering, Metallurgy, Materials Sci and Engn, Optics & Acoustics, Physics,
and Spectrosc/Instrum/Analyt Sci
Other
Engineering
Aerospace Engineering, Civil Engineering, Environmt Engineering/Energy,
Engineering Mgmt/General, Geol/Petrol/Mining Engn, Instrumentation/
Measurement, Nuclear Engineering, and Space Science
Other Sciences Aquatic Sciences, Earth Sciences, and Multidisciplinary
Source: Darby and Zucker (1999).